User:Hripsime Ashikyan
![]() | This article may be too technical for most readers to understand.(October 2011) |
![]() Mechanical interior of a modern hard disk drive | |
Date invented | 24 December 1954 [1] |
---|---|
Invented by | IBM team led by Rey Johnson |
A hard disk drive (HDD; also hard drive or hard disk)[2] is a non-volatile, random access digital magnetic data storage device. It features rotating rigid platters on a motor-driven spindle within a protective enclosure. Data is magnetically read from and written to the platter by read/write heads that float on a film of air above the platters. Introduced by IBM in 1956, hard disk drives have decreased in cost and physical size over the years while dramatically increasing in capacity.
Hard disk drives have been the dominant device for secondary storage of data in general purpose computers since the early 1960s.[3] They have maintained this position because advances in their recording density have kept pace with the requirements for secondary storage.[3] Today's HDDs operate on high-speed serial interfaces; i.e., serial ATA (SATA) or serial attached SCSI (SAS).
History[edit]
Hard disk drives were introduced in 1956 as data storage for an IBM real time transaction processing computer[4] and were developed for use with general purpose mainframe and mini computers.
As the 1980s began, hard disk drives were a rare and very expensive additional feature on personal computers (PCs); however by the late '80s, hard disk drives were standard on all but the cheapest PC.
Most hard disk drives in the early 1980s were sold to PC end users as an add on subsystem, not under the drive manufacturer's name but by systems integrators such as the Corvus Disk System or the systems manufacturer such as the Apple ProFile. The IBM PC/XT in 1983 included an internal standard 10MB hard disk drive, and soon thereafter internal hard disk drives proliferated on personal computers.
External hard disk drives remained popular for much longer on the Apple Macintosh. Every Mac made between 1986 and 1998 has a SCSI port on the back, making external expansion easy; also, "toaster" Compact Macs did not have easily accessible hard drive bays (or, in the case of the Mac Plus, any hard drive bay at all), so on those models, external SCSI disks were the only reasonable option.
Driven by areal density doubling every two to four years since their invention, HDDs have changed in many ways. A few highlights include:
- Capacity per HDD increasing from 3.75 megabytes to greater than 1 terabyte, a greater than 270-thousand-to-1 improvement.
- Size of HDD decreasing from 87.9 cubic feet (a double wide refrigerator) to 0.002 cubic feet (2½-inch form factor, a pack of cards), a greater than 44-thousand-to-1 improvement.
- Price decreasing from about $15,000 per megabyte to less than $0.0001 per megabyte ($100/1 terabyte), a greater than 150-million-to-1 improvement.[5]
- Average access time decreasing from greater than 0.1 second to a few thousandths of a second, a greater than 40-to-1 improvement.
- Market application expanding from general purpose computers to most computing applications including consumer applications.
Technology[edit]
Magnetic recording[edit]
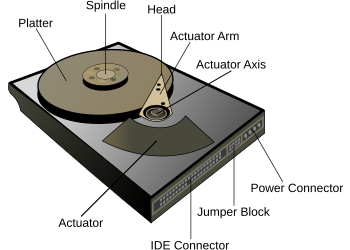
HDDs record data by magnetizing ferromagnetic material directionally. Sequential changes in the direction of magnetization represent patterns of binary data bits. The data are read from the disk by detecting the transitions in magnetization and decoding the originally written data. Different encoding schemes, such as modified frequency modulation, group code recording, run-length limited encoding, and others are used.
A typical HDD design consists of a spindle[6] that holds flat circular disks, also called platters, which hold the recorded data. The platters are made from a non-magnetic material, usually aluminum alloy, glass, or ceramic, and are coated with a shallow layer of magnetic material typically 10–20 nm in depth, with an outer layer of carbon for protection.[7][8][9] For reference, a standard piece of copy paper is 0.07–0.18 millimetres (70,000–180,000 nm).[10]



The platters in contemporary HDDs are spun at speeds varying from 4200 rpm in energy-efficient portable devices, to 15,000 rpm for high performance servers.[12] The first hard drives spun at 1200 rpm,[13] and for many years, 3600 rpm was the norm.[14] Information is written to, and read from a platter as it rotates past devices called read-and-write heads that operate very close (tens of nanometers in new drives) over the magnetic surface. The read-and-write head is used to detect and modify the magnetization of the material immediately under it. In modern drives there is one head for each magnetic platter surface on the spindle, mounted on a common arm. An actuator arm (or access arm) moves the heads on an arc (roughly radially) across the platters as they spin, allowing each head to access almost the entire surface of the platter as it spins. The arm is moved using a voice coil actuator or in some older designs a stepper motor.
The magnetic surface of each platter is conceptually divided into many small sub-micrometer-sized magnetic regions referred to as magnetic domains. In older disk designs the regions were oriented horizontally and parallel to the disk surface, but beginning about 2005, the orientation was changed to perpendicular to allow for closer magnetic domain spacing. Due to the polycrystalline nature of the magnetic material each of these magnetic regions is composed of a few hundred magnetic grains. Magnetic grains are typically 10 nm in size and each form a single magnetic domain. Each magnetic region in total forms a magnetic dipole which generates a magnetic field.
For reliable storage of data, the recording material needs to resist self-demagnetization, which occurs when the magnetic domains repel each other. Magnetic domains written too densely together to a weakly magnetizable material will degrade over time due to physical rotation of one or more domains to cancel out these forces. The domains rotate sideways to a halfway position that weakens the readability of the domain and relieves the magnetic stresses. Older hard disks used iron(III) oxide as the magnetic material, but current disks use a cobalt-based alloy.[15]
A write head magnetizes a region by generating a strong local magnetic field. Early HDDs used an electromagnet both to magnetize the region and to then read its magnetic field by using electromagnetic induction. Later versions of inductive heads included metal in Gap (MIG) heads and thin film heads. As data density increased, read heads using magnetoresistance (MR) came into use; the electrical resistance of the head changed according to the strength of the magnetism from the platter. Later development made use of spintronics; in these heads, the magnetoresistive effect was much greater than in earlier types, and was dubbed "giant" magnetoresistance (GMR). In today's heads, the read and write elements are separate, but in close proximity, on the head portion of an actuator arm. The read element is typically magneto-resistive while the write element is typically thin-film inductive.[16]
The heads are kept from contacting the platter surface by the air that is extremely close to the platter; that air moves at or near the platter speed. The record and playback head are mounted on a block called a slider, and the surface next to the platter is shaped to keep it just barely out of contact. This forms a type of air bearing.
In modern drives, the small size of the magnetic regions creates the danger that their magnetic state might be lost because of thermal effects. To counter this, the platters are coated with two parallel magnetic layers, separated by a 3-atom layer of the non-magnetic element ruthenium, and the two layers are magnetized in opposite orientation, thus reinforcing each other.[17] Another technology used to overcome thermal effects to allow greater recording densities is perpendicular recording, first shipped in 2005,[18] and as of 2007 the technology was used in many HDDs.[19][20][21]
Components[edit]

A typical hard disk drive has two electric motors; a disk motor that spins the disks and an actuator (motor) that positions the read/write head assembly across the spinning disks.
The disk motor has an external rotor attached to the disks; the stator windings are fixed in place.
Opposite the actuator at the end of the head support arm is the read-write head (near center in photo); thin printed-circuit cables connect the read-write heads to amplifier electronics mounted at the pivot of the actuator. A flexible, somewhat U-shaped, ribbon cable, seen edge-on below and to the left of the actuator arm continues the connection to the controller board on the opposite side.
The head support arm is very light, but also stiff; in modern drives, acceleration at the head reaches 550 g.
The silver-colored structure at the upper left of the first image is the top plate of the actuator, a permanent-magnet and moving coil motor that swings the heads to the desired position (it is shown removed in the second image). The plate supports a squat neodymium-iron-boron (NIB) high-flux magnet. Beneath this plate is the moving coil, often referred to as the voice coil by analogy to the coil in loudspeakers, which is attached to the actuator hub, and beneath that is a second NIB magnet, mounted on the bottom plate of the motor (some drives only have one magnet).

The voice coil itself is shaped rather like an arrowhead, and made of doubly coated copper magnet wire. The inner layer is insulation, and the outer is thermoplastic, which bonds the coil together after it is wound on a form, making it self-supporting. The portions of the coil along the two sides of the arrowhead (which point to the actuator bearing center) interact with the magnetic field, developing a tangential force that rotates the actuator. Current flowing radially outward along one side of the arrowhead and radially inward on the other produces the tangential force. If the magnetic field were uniform, each side would generate opposing forces that would cancel each other out. Therefore the surface of the magnet is half N pole, half S pole, with the radial dividing line in the middle, causing the two sides of the coil to see opposite magnetic fields and produce forces that add instead of canceling. Currents along the top and bottom of the coil produce radial forces that do not rotate the head.
Error handling[edit]
Modern drives also make extensive use of Error Correcting Codes (ECCs), particularly Reed–Solomon error correction. These techniques store extra bits for each block of data that are determined by mathematical formulas. The extra bits allow many errors to be fixed. While these extra bits take up space on the hard drive, they allow higher recording densities to be employed, resulting in much larger storage capacity for user data.[22] In 2009, in the newest drives, low-density parity-check codes (LDPC) are supplanting Reed-Solomon. LDPC codes enable performance close to the Shannon Limit and thus allow for the highest storage density available.[23]
Typical hard drives attempt to "remap" the data in a physical sector that is going bad to a spare physical sector—hopefully while the errors in that bad sector are still few enough that the ECC can recover the data without loss. The S.M.A.R.T. system counts the total number of errors in the entire hard drive fixed by ECC, and the total number of remappings, in an attempt to predict hard drive failure.
Future development[edit]
Due to bit-flipping errors and other issues, perpendicular recording densities may be supplanted by other magnetic recording technologies. Toshiba is promoting bit-patterned recording (BPR),[24] while Xyratex are developing heat-assisted magnetic recording (HAMR).[25]
October 2011: TDK has developed a special laser that heats up a hard's disk's surface with a precision of a few dozen nanometers. TDK also used the new material in the magnetic head and redesigned its structure to expand the recording density. This new technology apparently makes it possible to store one terabyte on one platter and for the initial. TDK will produce HDD with 2 platters.[26]
Capacity[edit]
The capacity of an HDD may appear to the end user to be a different amount than the amount stated by a drive or system manufacturer due to amongst other things, different units of measuring capacity, capacity consumed in formatting the drive for use by an operating system and/or redundancy.
Units of storage capacity[edit]
Advertised capacity by manufacturer (using decimal multiples) |
Expected capacity by consumers in class action (using binary multiples) |
Reported capacity | |||
---|---|---|---|---|---|
Windows (using binary multiples) |
Mac OS X 10.6+ (using decimal multiples) | ||||
With prefix | Bytes | Bytes | Diff. | ||
100 MB | 100,000,000 | 104,857,600 | 4.86% | 95.4 MB | 100.0 MB |
100 GB | 100,000,000,000 | 107,374,182,400 | 7.37% | 93.1 GB, 95,367 MB | 100.00 GB |
1 TB | 1,000,000,000,000 | 1,099,511,627,776 | 9.95% | 931 GB, 953,674 MB | 1000.00 GB, 1000,000 MB |
The capacity of hard disk drives is given by manufacturers in megabytes (1 MB = 1,000,000 bytes), gigabytes (1 GB = 1,000,000,000 bytes) or terabytes (1 TB = 1,000,000,000,000 bytes).[27][28] This numbering convention, where prefixes like mega- and giga- denote powers of 1000, is also used for data transmission rates and DVD capacities. However, the convention is different from that used by manufacturers of memory (RAM, ROM) and CDs, where prefixes like kilo- and mega- mean powers of 1024.
When the unit prefixes like kilo- denote powers of 1024 in the measure of memory capacities, the 1024n progression (for n = 1, 2, …) is as follows:[27]
- kilo = 210 = 10241 = 1024,
- mega = 220 = 10242 = 1,048,576,
- giga = 230 = 10243 = 1,073,741,824,
- tera = 240 = 10244 = 1,099,511,627,776,
and so forth.
The practice of using prefixes assigned to powers of 1000 within the hard drive and computer industries dates back to the early days of computing.[29] By the 1970s million, mega and M were consistently being used in the powers of 1000 sense to describe HDD capacity.[30][31][32] As HDD sizes grew the industry adopted the prefixes “G” for giga and “T” for tera denoting 1,000,000,000 and 1,000,000,000,000 bytes of HDD capacity respectively.
Likewise, the practice of using prefixes assigned to powers of 1024 within the computer industry also traces its roots to the early days of computing[33] By the early 1970s using the prefix “K” in a powers of 1024 sense to describe memory was common within the industry.[34][35] As memory sizes grew the industry adopted the prefixes “M” for mega and “G” for giga denoting 1,048,576 and 1,073,741,824 bytes of memory respectively.
Computers do not internally represent HDD or memory capacity in powers of 1024; reporting it in this manner is just a convention.[36] Creating confusion, operating systems report HDD capacity in different ways. Most operating systems, including the Microsoft Windows operating systems use the powers of 1024 convention when reporting HDD capacity, thus an HDD offered by its manufacturer as a 1 TB drive is reported by these OSes as a 931 GB HDD. Apple's current OSes, beginning with Mac OS X 10.6 (“Snow Leopard”), use powers of 1000 when reporting HDD capacity, thereby avoiding any discrepancy between what it reports and what the manufacturer advertises.
In the case of “mega-,” there is a nearly 5% difference between the powers of 1000 definition and the powers of 1024 definition. Furthermore, the difference is compounded by 2.4% with each incrementally larger prefix (gigabyte, terabyte, etc.) The discrepancy between the two conventions for measuring capacity was the subject of several class action suits against HDD manufacturers. The plaintiffs argued that the use of decimal measurements effectively misled consumers[37][38] while the defendants denied any wrongdoing or liability, asserting that their marketing and advertising complied in all respects with the law and that no Class Member sustained any damages or injuries.[39]
In December 1998, an international standards organization attempted to address these dual definitions of the conventional prefixes by proposing unique binary prefixes and prefix symbols to denote multiples of 1024, such as “mebibyte (MiB)”, which exclusively denotes 220 or 1,048,576 bytes.[40] In the over‑25 years that have since elapsed, the proposal has seen little adoption by the computer industry and the conventionally prefixed forms of “byte” continue to denote slightly different values depending on context.[41][42]
HDD formatting[edit]
The presentation of an HDD to its host is determined by its controller. This may differ substantially from the drive's native interface particularly in mainframes or servers.
Modern HDDs, such as SAS[43] and SATA[44] drives, appear at their interfaces as a contiguous set of logical blocks; typically 512 bytes long but the industry is in the process of changing to 4,096 byte logical blocks; see Advanced Format.[45]
The process of initializing these logical blocks on the physical disk platters is called low level formatting which is usually performed at the factory and is not normally changed in the field.[46]
High level formatting then writes the file system structures into selected logical blocks to make the remaining logical blocks available to the host OS and its applications.[47] The operating system file system uses some of the disk space to organize files on the disk, recording their file names and the sequence of disk areas that represent the file. Examples of data structures stored on disk to retrieve files include the MS DOS file allocation table (FAT), and UNIX inodes, as well as other operating system data structures. As a consequence not all the space on a hard drive is available for user files. This file system overhead is usually less than 1% on drives larger than 100 MB.
Redundancy[edit]
In modern HDDs spare capacity for defect management is not included in the published capacity; however in many early HDDs a certain number of sectors were reserved for spares, thereby reducing capacity available to end users.
In some systems, there may be hidden partitions used for system recovery that reduce the capacity available to the end user.
For RAID drives, data integrity and fault-tolerance requirements also reduce the realized capacity. For example, a RAID1 drive will be about half the total capacity as a result of data mirroring. For RAID5 drives with x drives you would lose 1/x of your space to parity. RAID drives are multiple drives that appear to be one drive to the user, but provides some fault-tolerance. Most RAID vendors use some form of checksums to improve data integrity at the block level. For many vendors, this involves using HDDs with sectors of 520 bytes per sector to contain 512 bytes of user data and 8 checksum bytes or using separate 512 byte sectors for the checksum data.[48]
HDD parameters to calculate capacity[edit]
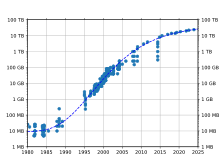
Because modern disk drives appear to their interface as a contiguous set of logical blocks their gross capacity can be calculated by multiplying the number of blocks by the size of the block. This information is available from the manufacturers specification and from the drive itself through use of special utilities invoking low level commands[43][44]
The gross capacity of older HDDs can be calculated by multiplying for each zone of the drive the number of cylinders by the number of heads by the number of sectors/zone by the number of bytes/sector (most commonly 512) and then summing the totals for all zones. Some modern ATA drives will also report cylinder, head, sector (C/H/S) values to the CPU but they are no longer actual physical parameters since the reported numbers are constrained by historic operating-system interfaces.
The old C/H/S scheme has been replaced by logical block addressing. In some cases, to try to "force-fit" the C/H/S scheme to large-capacity drives, the number of heads was given as 64, although no modern drive has anywhere near 32 platters.
Form factors[edit]
2½″ (8.5 mm) 6495 MB HDD

Mainframe and minicomputer hard disks were of widely varying dimensions, typically in free standing cabinets the size of washing machines (e.g. HP 7935 and DEC RP06 Disk Drives) or designed so that dimensions enabled placement in a 19" rack (e.g. Diablo Model 31). In 1962, IBM introduced its model 1311 disk, which used 14 inch (nominal size) platters. This became a standard size for mainframe and minicomputer drives for many years,[49] but such large platters were never used with microprocessor-based systems.
With increasing sales of microcomputers having built in floppy-disk drives (FDDs), HDDs that would fit to the FDD mountings became desirable, and this led to the evolution of the market towards drives with certain Form factors, initially derived from the sizes of 8-inch, 5.25-inch, and 3.5-inch floppy disk drives. Smaller sizes than 3.5 inches have emerged as popular in the marketplace and/or been decided by various industry groups.
- 8 inch: 9.5 in × 4.624 in × 14.25 in (241.3 mm × 117.5 mm × 362 mm)
In 1979, Shugart Associates' SA1000 was the first form factor compatible HDD, having the same dimensions and a compatible interface to the 8″ FDD. - 5.25 inch: 5.75 in × 3.25 in × 8 in (146.1 mm × 82.55 mm × 203 mm)
This smaller form factor, first used in an HDD by Seagate in 1980,[50] was the same size as full-height 5+1⁄4-inch-diameter (130 mm) FDD, 3.25-inches high. This is twice as high as "half height"; i.e., 1.63 in (41.4 mm). Most desktop models of drives for optical 120 mm disks (DVD, CD) use the half height 5¼″ dimension, but it fell out of fashion for HDDs. The Quantum Bigfoot HDD was the last to use it in the late 1990s, with "low-profile" (≈25 mm) and "ultra-low-profile" (≈20 mm) high versions. - 3.5 inch: 4 in × 1 in × 5.75 in (101.6 mm × 25.4 mm × 146 mm) = 376.77344 cm³
This smaller form factor is similar to that used in an HDD by Rodime in 1983,[51], which was the same size as the "half height" 3½″ FDD, i.e., 1.63 inches high. Today, the 1-inch high ("slimline" or "low-profile") version of this form factor is the most popular form used in most desktops. - 2.5 inch: 2.75 in × 0.275–0.59 in × 3.945 in (69.85 mm × 7–15 mm × 100 mm) = 48.895–104.775 cm3
This smaller form factor was introduced by PrairieTek in 1988;[52] there is no corresponding FDD. It is widely used today for solid-state drives and for hard disk drives in mobile devices (laptops, music players, etc.) and as of 2008 replacing 3.5 inch enterprise-class drives.[53] It is also used in the Playstation 3[54] and Xbox 360[citation needed] video game consoles. Today, the dominant height of this form factor is 9.5 mm for laptop drives (usually having two platters inside), but higher capacity drives have a height of 12.5 mm (usually having three platters). Enterprise-class drives can have a height up to 15 mm.[55] Seagate released a 7mm drive aimed at entry level laptops and high end netbooks in December 2009.[56] - 1.8 inch: 54 mm × 8 mm × 71 mm = 30.672 cm³
This form factor, originally introduced by Integral Peripherals in 1993, has evolved into the ATA-7 LIF with dimensions as stated. It was increasingly used in digital audio players and subnotebooks, but is rarely used today. An original variant exists for 2–5GB sized HDDs that fit directly into a PC card expansion slot. These became popular for their use in iPods and other HDD based MP3 players. - 1 inch: 42.8 mm × 5 mm × 36.4 mm
This form factor was introduced in 1999 as IBM's Microdrive to fit inside a CF Type II slot. Samsung calls the same form factor "1.3 inch" drive in its product literature.[57] - 0.85 inch: 24 mm × 5 mm × 32 mm
Toshiba announced this form factor in January 2004[58] for use in mobile phones and similar applications, including SD/MMC slot compatible HDDs optimized for video storage on 4G handsets. Toshiba currently sells a 4 GB (MK4001MTD) and 8 GB (MK8003MTD) version [1][dead link] and holds the Guinness World Record for the smallest hard disk drive.[59]
3.5-inch and 2.5-inch hard disks currently dominate the market.
By 2009 all manufacturers had discontinued the development of new products for the 1.3-inch, 1-inch and 0.85-inch form factors due to falling prices of flash memory,[60][61] which is slightly more stable and resistant to damage from impact and/or dropping.
The inch-based nickname of all these form factors usually do not indicate any actual product dimension (which are specified in millimeters for more recent form factors), but just roughly indicate a size relative to disk diameters, in the interest of historic continuity.
Current hard disk form factors[edit]
Form factor | Width (mm) | Height (mm) | Largest capacity | Platters (Max) |
---|---|---|---|---|
3.5″ | 102 | 19 or 25.4 | 4 TB[62][63][64][65] (2011) | 5 |
2.5″ | 69.9 | 7[66], 9.5[67], 11.5[68], or 15 | 1.5 TB[62][69][70][71] (2010) | 4 |
1.8″ | 54 | 5 or 8 | 320 GB[72] (2009) | 3 |
Obsolete hard disk form factors[edit]
Form factor | Width (mm) | Largest capacity | Platters (Max) |
---|---|---|---|
5.25″ FH | 146 | 47 GB[73] (1998) | 14 |
5.25″ HH | 146 | 19.3 GB[74] (1998) | 4[75] |
1.3″ | 43 | 40 GB[76] (2007) | 1 |
1″ (CFII/ZIF/IDE-Flex) | 42 | 20 GB (2006) | 1 |
0.85″ | 24 | 8 GB[77][78] (2004) | 1 |
Performance characteristics[edit]
Access time[edit]
The factors that limit the time to access the data on a hard disk drive (Access time) are mostly related to the mechanical nature of the rotating disks and moving heads. Seek time is a measure of how long it takes the head assembly to travel to the track of the disk that contains data. Rotational latency is incurred because the desired disk sector may not be directly under the head when data transfer is requested. These two delays are on the order of milliseconds each. The bit rate or data transfer rate once the head is in the right position creates delay which is a function of the number of blocks transferred; typically relatively small, but can be quite long with the transfer of large contiguous files. Delay may also occur if the drive disks are stopped to save energy, see Power management.
An HDD's Average Access Time is its average Seek time which technically is the time to do all possible seeks divided by the number of all possible seeks, but in practice is determined by statistical methods or simply approximated as the time of a seek over one-third of the number of tracks[79]
Defragmentation is a procedure used to minimize delay in retrieving data by moving related items to physically proximate areas on the disk.[80] Some computer operating systems perform defragmentation automatically. Although automatic defragmentation is intended to reduce access delays, the procedure can slow response when performed while the computer is in use.[81]
Access time can be improved by increasing rotational speed, thus reducing latency and/or by decreasing seek time. Increasing areal density increases throughput by increasing data rate and by increasing the amount of data under a set of heads, thereby potentially reducing seek activity for a given amount of data. Based on historic trends, analysts predict a future growth in HDD areal density (and therefore capacity) of about 40% per year.[82] Access times have not kept up with throughput increases, which themselves have not kept up with growth in storage capacity.
Interleave[edit]
[[:Image:IBM PC XT 10 meg MFM low level format.jpg|thumb|200px|Low-level formatting software to find highest performance interleave choice for 10 MB IBM PC XT hard disk drive.]]
Sector interleave is a mostly obsolete device characteristic related to access time, dating back to when computers were too slow to be able to read large continuous streams of data. Interleaving introduced gaps between data sectors to allow time for slow equipment to get ready to read the next block of data. Without interleaving, the next logical sector would arrive at the read/write head before the equipment was ready, requiring the system to wait for another complete disk revolution before reading could be performed.
However, because interleaving introduces intentional physical delays into the drive mechanism, setting the interleave to a ratio higher than required causes unnecessary delays for equipment that has the performance needed to read sectors more quickly. The interleaving ratio was therefore usually chosen by the end-user to suit their particular computer system's performance capabilities when the drive was first installed in their system.
Modern technology is capable of reading data as fast as it can be obtained from the spinning platters, so hard drives usually have a fixed sector interleave ratio of 1:1, which is effectively no interleaving being used.
Seek time[edit]
Average seek time ranges from 3 ms[83] for high-end server drives, to 15 ms for mobile drives, with the most common mobile drives at about 12 ms[84] and the most common desktop type typically being around 9 ms. The first HDD had an average seek time of about 600 ms and by the middle 1970s HDDs were available with seek times of about 25 ms. Some early PC drives used a stepper motor to move the heads, and as a result had seek times as slow as 80–120 ms, but this was quickly improved by voice coil type actuation in the 1980s, reducing seek times to around 20 ms. Seek time has continued to improve slowly over time.
Some desktop and laptop computer systems allow the user to make a tradeoff between seek performance and drive noise. Faster seek rates typically require more energy usage to quickly move the heads across the platter, causing loud noises from the pivot bearing and greater device vibrations as the heads are rapidly accelerated during the start of the seek motion and decelerated at the end of the seek motion. Quiet operation reduces movement speed and acceleration rates, but at a cost of reduced seek performance.
Rotational latency[edit]
Rotational speed [rpm] |
Average latency [ms] |
---|---|
15000 | 2 |
10000 | 3 |
7200 | 4.16 |
5400 | 5.55 |
4800 | 6.25 |
Latency is the delay for the rotation of the disk to bring the required disk sector under the read-write mechanism. It depends on rotational speed of a disk, measured in revolutions per minute (rpm). Average rotational latency is shown in the table below, based on the empirical relation that the average latency in milliseconds for such a drive is one-half the rotational period.
Data transfer rate[edit]
As of 2010, a typical 7200 rpm desktop hard drive has a sustained "disk-to-buffer" data transfer rate up to 1030 Mbits/sec.[85] This rate depends on the track location, so it will be higher for data on the outer tracks (where there are more data sectors) and lower toward the inner tracks (where there are fewer data sectors); and is generally somewhat higher for 10,000 rpm drives. A current widely used standard for the "buffer-to-computer" interface is 3.0 Gbit/s SATA, which can send about 300 megabyte/s (10 bit encoding) from the buffer to the computer, and thus is still comfortably ahead of today's disk-to-buffer transfer rates. Data transfer rate (read/write) can be measured by writing a large file to disk using special file generator tools, then reading back the file. Transfer rate can be influenced by file system fragmentation and the layout of the files.[80]
HDD data transfer rate depends upon the rotational speed of the platters and the data recording density. Because heat and vibration limit rotational speed, advancing density becomes the main method to improve sequential transfer rates.[citation needed] While areal density advances by increasing both the number of tracks across the disk and the number of sectors per track, only the latter will increase the data transfer rate for a given rpm. Since data transfer rate performance only tracks one of the two components of areal density, its performance improves at a lower rate.
Power consumption[edit]
Power consumption has become increasingly important, not only in mobile devices such as laptops but also in server and desktop markets. Increasing data center machine density has led to problems delivering sufficient power to devices (especially for spin up), and getting rid of the waste heat subsequently produced, as well as environmental and electrical cost concerns (see green computing). Heat dissipation is tied directly to power consumption, and as drives age, disk failure rates increase at higher drive temperatures.[86] Similar issues exist for large companies with thousands of desktop PCs. Smaller form factor drives often use less power than larger drives. One interesting development in this area is actively controlling the seek speed so that the head arrives at its destination only just in time to read the sector, rather than arriving as quickly as possible and then having to wait for the sector to come around (i.e. the rotational latency).[87] Many of the hard drive companies are now producing Green Drives that require much less power and cooling. Many of these Green Drives spin slower (<5400 rpm compared to 7200, 10,000 or 15,000 rpm) thereby generating less heat. Power consumption can also be reduced by parking the drive heads when the disk is not in use reducing friction, adjusting spin speeds,[88] and disabling internal components when not in use.[89]
Also in systems where there might be multiple hard disk drives, there are various ways of controlling when the hard drives spin up since the highest current is drawn at that time.
- On SCSI hard disk drives, the SCSI controller can directly control spin up and spin down of the drives.
- On Parallel ATA (PATA) and Serial ATA (SATA) hard disk drives, some support power-up in standby or PUIS. The hard disk drive will not spin up until the controller or system BIOS issues a specific command to do so. This limits the power draw or consumption upon power on.
- Some SATA II hard disk drives support Spin-up#Staggered_spin-up, allowing the computer to spin up the drives in sequence to reduce load on the power supply when booting.[90]
Power management[edit]
Most hard disk drives today support some form of power management which uses a number of specific power modes that save energy by reducing performance. When implemented an HDD will change between a full power mode to one or more power saving modes as a function of drive usage. Recovery from the deepest mode, typically called Sleep, may take as long as several seconds.[91]
Audible noise[edit]
Measured in dBA, audible noise is significant for certain applications, such as DVRs, digital audio recording and quiet computers. Low noise disks typically use fluid bearings, slower rotational speeds (usually 5400 rpm) and reduce the seek speed under load (AAM) to reduce audible clicks and crunching sounds. Drives in smaller form factors (e.g. 2.5 inch) are often quieter than larger drives.
Shock resistance[edit]
Shock resistance is especially important for mobile devices. Some laptops now include active hard drive protection that parks the disk heads if the machine is dropped, hopefully before impact, to offer the greatest possible chance of survival in such an event. Maximum shock tolerance to date is 350 g for operating and 1000 g for non-operating.[92]
Access and interfaces[edit]
This section needs additional citations for verification. (July 2009) |
Hard disk drives are accessed over one of a number of bus types, including parallel ATA (PATA, also called IDE or EIDE), Serial ATA (SATA), SCSI, Serial Attached SCSI (SAS), and Fibre Channel. Bridge circuitry is sometimes used to connect hard disk drives to buses with which they cannot communicate natively, such as IEEE 1394, USB and SCSI.
For the ST-506 interface, the data encoding scheme as written to the disk surface was also important. The first ST-506 disks used Modified Frequency Modulation (MFM) encoding, and transferred data at a rate of 5 megabits per second. Later controllers using 2,7 RLL (or just "RLL") encoding caused 50% more data to appear under the heads compared to one rotation of an MFM drive, increasing data storage and data transfer rate by 50%, to 7.5 megabits per second.
Many ST-506 interface disk drives were only specified by the manufacturer to run at the 1/3 lower MFM data transfer rate compared to RLL, while other drive models (usually more expensive versions of the same drive) were specified to run at the higher RLL data transfer rate. In some cases, a drive had sufficient margin to allow the MFM specified model to run at the denser/faster RLL data transfer rate (not recommended nor guaranteed by manufacturers). Also, any RLL-certified drive could run on any MFM controller, but with 1/3 less data capacity and as much as 1/3 less data transfer rate compared to its RLL specifications.
Enhanced Small Disk Interface (ESDI) also supported multiple data rates (ESDI disks always used 2,7 RLL, but at 10, 15 or 20 megabits per second), but this was usually negotiated automatically by the disk drive and controller; most of the time, however, 15 or 20 megabit ESDI disk drives were not downward compatible (i.e. a 15 or 20 megabit disk drive would not run on a 10 megabit controller). ESDI disk drives typically also had jumpers to set the number of sectors per track and (in some cases) sector size.
Modern hard drives present a consistent interface to the rest of the computer, no matter what data encoding scheme is used internally. Typically a DSP in the electronics inside the hard drive takes the raw analog voltages from the read head and uses PRML and Reed–Solomon error correction[93] to decode the sector boundaries and sector data, then sends that data out the standard interface. That DSP also watches the error rate detected by error detection and correction, and performs bad sector remapping, data collection for Self-Monitoring, Analysis, and Reporting Technology, and other internal tasks.
SCSI originally had just one signaling frequency of 5 MHz for a maximum data rate of 5 megabytes/second over 8 parallel conductors, but later this was increased dramatically. The SCSI bus speed had no bearing on the disk's internal speed because of buffering between the SCSI bus and the disk drive's internal data bus; however, many early disk drives had very small buffers, and thus had to be reformatted to a different interleave (just like ST-506 disks) when used on slow computers, such as early Commodore Amiga, IBM PC compatibles and Apple Macintoshes.
ATA disks have typically had no problems with interleave or data rate, due to their controller design, but many early models were incompatible with each other and could not run with two devices on the same physical cable in a master/slave setup. This was mostly remedied by the mid-1990s, when ATA's specification was standardized and the details began to be cleaned up, but still causes problems occasionally (especially with CD-ROM and DVD-ROM disks, and when mixing Ultra DMA and non-UDMA devices).
Serial ATA does away with master/slave setups entirely, placing each disk on its own channel (with its own set of I/O ports) instead.
FireWire/IEEE 1394 and USB(1.0/2.0/3.0) hard drives consist of enclosures containing generally ATA or Serial ATA disks with built-in adapters to these external buses.
Disk interface families used in personal computers[edit]

Historical bit serial interfaces connect a hard disk drive (HDD) to a hard disk controller (HDC) with two cables, one for control and one for data. (Each drive also has an additional cable for power, usually connecting it directly to the power supply unit). The HDC provided significant functions such as serial/parallel conversion, data separation, and track formatting, and required matching to the drive (after formatting) in order to assure reliability. Each control cable could serve two or more drives, while a dedicated (and smaller) data cable served each drive.
- ST506 used MFM (Modified Frequency Modulation) for the data encoding method.
- ST412 was available in either MFM or RLL (Run Length Limited) encoding variants.
- Enhanced Small Disk Interface (ESDI) was an industry standard interface similar to ST412 supporting higher data rates between the processor and the disk drive.
Modern bit serial interfaces connect a hard disk drive to a host bus interface adapter (today typically integrated into the "south bridge") with one data/control cable. (As for historical bit serial interfaces above, each drive also has an additional power cable, usually direct to the power supply unit.)
- Fibre Channel (FC) is a successor to parallel SCSI interface on enterprise market. It is a serial protocol. In disk drives usually the Fibre Channel Arbitrated Loop (FC-AL) connection topology is used. FC has much broader usage than mere disk interfaces, and it is the cornerstone of storage area networks (SANs). Recently other protocols for this field, like iSCSI and ATA over Ethernet have been developed as well. Confusingly, drives usually use copper twisted-pair cables for Fibre Channel, not fibre optics. The latter are traditionally reserved for larger devices, such as servers or disk array controllers.
- Serial ATA (SATA). The SATA data cable has one data pair for differential transmission of data to the device, and one
- ^ This is the original filing date of the application which led to US Patent 3,503,060, generally accepted as the definitive disk drive patent; see, Kean, David W., "IBM San Jose, A quarter century of innovation", 1977.
- ^ Further terms used to describe hard disk drives include disk drive, disk file, direct access storage device (DASD), fixed disk, CKD disk, and Winchester Disk Drive (after the IBM 3340). The term DASD includes other devices besides disks.
- ^ a b Magnetic Storage Handbook 2nd Ed., Section 2.1.1, Disk File Technology, Mee and Daniel, (c)1990,
- ^ "IBM 350 disk storage unit". IBM. Retrieved 24 June 2010.
- ^ Scotia, Nova. "Cost of Hard Drive storage Space". Retrieved 12 March 2011.
- ^ In storage engineering, the term spindle also refers to a single drive that can only handle one or a limited number of I/O operations making it a point of focus in scheduling operations to an array of drives.
- ^ "Hard Drives". escotal.com. Retrieved 16 July 2011.
- ^ "What is a "head-crash" & how can it result in permanent loss of my hard drive data?". data-master.com. Retrieved 16 July 2011.
- ^ "Hard Drive Help". hardrivehelp.com. Retrieved 16 July 2011.
- ^ Elert, Glenn. "Thickness of a Piece of Paper". HyperTextbook.com. Retrieved 9 July 2011.
- ^ CMOS-MagView is an instrument that visualizes magnetic field structures and strengths.
- ^ Blount, Walker C. (November 2007). "Why 7200 RPM Mobile Hard Disk Drives?" (PDF). Retrieved 17 July 2011. The source states 3600 RPM drives were available in the past, but as of November 2007, 4200 was the lowest available from Hitachi.
- ^ See History of IBM magnetic disk drives
- ^ www.pcguide.com/ref/hdd/op/spin_Speed.htm
- ^ Kanellos, Michael (24 August 2006). "A divide over the future of hard drives". CNETNews.com. Retrieved 24 June 2010.
- ^ "IBM OEM MR Head | Technology | The era of giant magnetoresistive heads". Hitachigst.com. 27 August 2001. Retrieved 4 September 2010.
- ^ Brian Hayes, Terabyte Territory, American Scientist, Vol 90 No 3 (May–June 2002) p. 212
- ^ "Press Releases December 14, 2004". Toshiba. Retrieved 13 March 2009.
- ^ "Seagate Momentus 2½" HDDs per webpage Jan 2008". Seagate.com. 24 October 2008. Retrieved 13 March 2009.
- ^ "Seagate Barracuda 3½" HDDs per webpage January 2008". Seagate.com. Retrieved 13 March 2009.
- ^ "Western Digital Scorpio 2½" and Greenpower 3½" HDDs per quarterly conference, July 2007". Wdc.com. Retrieved 13 March 2009.
- ^ Error Correcting Code, Storage Review
- ^ "Iterative Detection Read Channel Technology in Hard Disk Drives", Hitachi
- ^ "Will Toshiba's Bit-Patterned Drives Change the HDD Landscape?". PC Magazine. 19 August 2010. Retrieved 21 August 2010.
- ^ "Xyratex no-go for bit-patterned media". The Register. 24 April 2010. Retrieved 21 August 2010.
- ^ "Report: TDK Technology "More Than Doubles" Capacity Of HDDs". Retrieved 4 October 2011.
- ^ a b Drive displays a smaller capacity than the indicated size on the drive label Cite error: The named reference "WD" was defined multiple times with different content (see the help page).
- ^ i.e. see HGST, Samsung, Seagate, Toshiba and Western Digital websites
- ^ "650 RAMAC announcement".IBM Press Release, 13 September 1956, announcing the IBM 305 RAMAC. The first disk drive invented by IBM in 1956 was described by IBM using the descriptive noun million, as in "... a built-in 5-million-digit disk memory"
- ^ Mulvany, R.B., "Engineering Design of a Disk Storage Facility with Data Modules." IBM JRD, November 1974
- ^ Introduction to IBM Direct Access Storage Devices, M. Bohl, IBM publication SR20-4738 ©1981.
- ^ CDC Product Line Card, October 1974
- ^ G. M. Amdahl; et al. (1964). "Architecture of the IBM System/360". IBM JRD. 8 (2).
Capacity 8 bit Bytes – 1K = 1024
{{cite journal}}
: Explicit use of et al. in:|author=
(help) - ^ IBM System/360 Operating System: Storage Estimates, 12th ed. (PDF), IBM Corp., 1971, p. 21, GC28-6551-12,
Model 40 with 64K bytes of storage and storage protection
{{citation}}
: Unknown parameter|month=
ignored (help) This document contains approximately 468 usages of K meaning 1024 - ^ PDP11/05/10/30/40 Processor Handbook (PDF), Digital Equipment Corp., 1973, p. 1-1,
... direct addressing of 32K 16-bit words or 64K 8-bit bytes (K = 1024)
This document contains approximately 80 instances of K meaning 1024. - ^ Topher Kessler. "Snow Leopard changes how file and drive sizes are calculated". cnet Reviews. "Altering this convention to agree with English could have been done at any time; however, for some reason it just stuck this way for a lot of the computing industry".
- ^ Western Digital Settles Hard-Drive Capacity Lawsuit, Associated Press 28 June 2006 retrieved 2010 Nov 25
- ^ Seagate lawsuit concludes, settlement announced
- ^ Western Digital – Notice of Class Action Settlement email
- ^ National Institute of Standards and Technology. "Prefixes for binary multiples". "In December 1998 the International Electrotechnical Commission (IEC) [...] approved as an IEC International Standard names and symbols for prefixes for binary multiples for use in the fields of data processing and data transmission."
- ^ Upgrading and Repairing PCs, Scott Mueller, Pg. 596, ISBN 0789729741
- ^ The silicon web: physics for the Internet age, Michael G. Raymer, Pg. 40, ISBN 9781439803110
- ^ a b
The LBAs on a logical unit shall begin with zero and shall be contiguous up to the last logical block on the logical unit.
— Information technology – Serial Attached SCSI – 2 (SAS-2), INCITS 457 Draft 2, 8 May 2009, chapter 4.1 Direct-access block device type model overview - ^ a b ISO/IEC 791D:1994, AT Attachment Interface for Disk Drives (ATA-1), section 7.1.2
- ^ Western Digital's Advanced Format: The 4K Sector Transition Begins
- ^ See: Low-Level Formatting. However, some enterprise SAS drives (e.g. Seagate Constellation ES) have other block sizes such as 520, 524 and 528 bytes which can be changed in the field.
- ^ High-Level Formatting
- ^ How to Measure Storage Efficiency – Part II – Taxes
- ^ Emerson W. Pugh, Lyle R. Johnson, John H. Palmer IBM's 360 and early 370 systems MIT Press, 1991 ISBN 0262161230, page 266
- ^ Christensen, Clayton M. (1997). The Innovator's Dilemma. New York, New York: HarperBusiness. p. 19. ISBN 0-06-662069-4.
{{cite book}}
: More than one of|pages=
and|page=
specified (help) - ^ "Winchester has 3.5-inch diameter". Electronics: 184. March 1983.
- ^ Chandler, Doug (26 September 1988). "Startup Ships 2.5-Inch Hard Disk Aimed for Portables, Laptops". PC Week: 6.
- ^ schmid, Patrick and Achim Roos (8 May 2010). "3.5" Vs. 2.5" SAS HDDs: In Storage, Size Matters". Tomshardware.com. Retrieved 25 June 2010.
- ^ "Playstation 3 Slim Teardown". 25 August 2009. Retrieved 15 November 2010.
- ^ Schmid, Patrick and Achim Roos (22 May 2010). "9.5 Versus 12.5 mm: Which Notebook HDD Is Right For You?". Tomshardware.com. Retrieved 22 June 2010.
- ^ "Seagate Unveils World's Thinnest 2.5-Inch Hard Drive For Slim Laptop Computers". physorg.com. 15 December 2009. Retrieved 15 December 2009.
- ^ 1.3″ HDD Product Specification, Samsung, 2008
- ^ Toshiba's 0.85-inch HDD is set to bring multi-gigabyte capacities to small, powerful digital products, Toshiba press release, 8 January 2004
- ^ Toshiba enters Guinness World Records Book with the world's smallest hard disk drive, Toshiba press release, 16 March 2004
- ^ Flash price fall shakes HDD market, EETimes Asia, 1 August 2007.
- ^ In 2008 Samsung introduced the 1.3-inch SpinPoint A1 HDD but by March 2009 the family was listed as End Of Life Products and new new 1.3-inch models were not available in this size.
- ^ a b Branded external only.
- ^ "Seagate's 4TB GoFlex Breaks the Hard Drive Capacity Ceiling". 7 September 2011. Retrieved 7 September 2011.
- ^ Seagate FreeAgent GoFlex 4TB Desk External Drive Review
- ^ 750 GB for IDE-based barebone, 3 TB for SATA-based barebone.
- ^ Proving that 2.5 inch hard disk drive with height of 7 mm exists.
- ^ Most common.
- ^ 750 GB, 1 TB, or 1.5 TB only.
- ^ http://www.engadget.com/photos/seagate-1-5tb-goflex-usb-3-0-external-hard-drive/#3374569/
- ^ http://www.pcworld.idg.com.au/review/servers_storage/seagate/freeagent_goflex_ultra-portable_drive_1_5tb/362043
- ^ 320 GB for IDE-based barebone, 1 TB for SATA-based barebone.
- ^ "Toshiba Storage Solutions – MK3233GSG".
- ^ Seagate Elite 47, shipped 12/97 per 1998 Disk/Trend Report – Rigid Disk Drives
- ^ Quantum Bigfoot TS, shipped 10/98 per 1999 Disk/Trend Report – Rigid Disk Drives
- ^ The Quantum Bigfoot TS used a maximum of 3 platters, other earlier and lower capacity product used up to 4 platters in a 5.25″ HH form factor, e.g. Microscience HH1090 circa 1989.
- ^ "SDK Starts Shipments of 1.3-Inch PMR-Technology-Based HD Media". Sdk.co.jp. 10 January 2008. Retrieved 13 March 2009.
- ^ Proving that 8 GB, 0.85 inch hard disk drive exists.
- ^ Toshiba Enters Guinness World Records Book with the World's Smallest Hard Disk Drive.
- ^ Western Digital definition of average access time
- ^ a b Kearns, Dave (18 April 2001). "How to defrag". ITWorld.
- ^ Broida, Rick (10 April 2009). "Turning Off Disk Defragmenter May Solve a Sluggish PC". PCWorld.
- ^ "Seagate Outlines the Future of Storage :: Articles :: www.hardwarezone.com". hardwarezone.com. 27 January 2006. Retrieved 13 March 2009.[dead link]
- ^ "WD VelicoRaptor: Drive Specifications". Western Digital. June 2010. Retrieved 15 January 2011.
- ^ "WD Scorpio Blue Mobile: Drive Specifications". Western Digital. June 2010. Retrieved 15 January 2011.
- ^ "Speed Considerations", Seagate website. Retrieved 22 January 2011.
- ^ Artamonov, Oleg (6 December 2007). "Hard Disk Drive Power Consumption Measurements: X-bit's Methodology". Xbit Laboratories.
- ^ e.g. Western Digital's Intelliseek
- ^ Hitachi Unveils Energy-Efficient Hard Drive with Variable Spindle Speed.
- ^ Webber, Lawrence; Wallace, Michael (2009). Green tech: how to plan and implement sustainable IT solutions. p. 62. ISBN 081441446X.
- ^ Trusted Reviews (31 August 2005). "Hitachi Deskstar 7K500 500GB HDD: As fast as it's big?".
- ^ Adaptive Power Management for Mobile Hard Drives
- ^ Momentus 5400.5 SATA 3Gb/s 320-GB Hard Drive
- ^ "Reed Solomon Codes – Introduction"